Hat is a Possible Longterm Effect of Continued Exposure to Air Pollution
Introduction
Air pollution poses a serious global threat to public health1,2,3,4,5. According to World Health Organization (WHO), around 4.2 million deaths per year are due to ambient air pollution, and about 91% of the world's population lives in areas where air quality levels are over WHO guidelines. Particulate matter (PM), ozone (O3), nitrogen dioxide (NO2), and sulfur dioxide (SO2) have been reported to endanger health and jointly constitute the dimensions of WHO air quality assessment.
Due to the rise in industrialization in the past decades, such as the growing steel casting, coal burning, oil extraction, and vehicle production, environmental pollution has increased in China. NO2 is produced by incomplete combustion inside motor engines, and traffic emission may be the primary source1,2. PM2.5 (PM with a diameter ≤ 2.5 µm) is part of PM10 (PM with a diameter ≤ 10 µm), and both of them originate from a variety of sources, including combustion, photochemical smog reactions, and sandstorms1,2. In China, people benefit from a high level of social development but suffer from the adverse health effects associated with air pollution. These pollutants are attributed to an extensive range of cardiovascular diseases3,4,5,6,7,8, pulmonary diseases9, metabolic diseases10, strokes11, and even sudden infant death syndrome12.
Increasing organ impairment has been associated with air pollution, and the ocular surface is not an exception since it is constantly exposed to the external environment. Symptoms of ocular surface pathologies tend to increase in a poor air environment, manifesting as irritation, foreign body sensation, redness, and blurred vision, among others13. Air pollutants have been shown to have adverse effects on dry eye disease (DED)13,14,15. Many studies have been conducted to investigate the changes in tear stability, tear volume, and corneal fluorescein staining (CFS) after exposure to air pollution. Chronic inflammation, allergy, and oxidative stress are involved in the pathogenesis of ocular surface diseases after air pollution exposure13. However, limited studies have assessed the changes in eyelid margin and meibomian gland dysfunction (MGD), which are supposed to exist before clinical manifestations are observed. The eyelid margin and MGD play key roles in the development of evaporative dry eye (DE). Additional, different regions may produce different pollutants and result in different ocular surface injury, which has not been mentioned in previous studies.
Because of the paucity of evidence regarding the effects of air pollutants on MGD in different locations in China, this study aimed to assess the changes in the ocular surface, particularly the lid margin, meibomian gland (MG) and tear cytokines, upon exposure to different air pollutants across different regions.
Materials and methods
Study design and participants
In this multicenter prospective cohort study, individuals were recruited from five provinces across China, including 11 hospitals in Beijing, Hebei, Heilongjiang, Anhui, and Inner Mongolia from February 2020 to February 2021, covering four different regions where factories mainly dedicated to steel, coal or oil production, and densely population (living) are predominant in the area. Participants aged 20–80 years who spend 3–4 h outdoor activities per day (average) in the corresponding zone and met diagnostic criteria of MGD16,17 were recruited in the study. Individuals with histories of another ocular surface abnormality, contact lens use, ocular surgeries, glaucoma medications use were excluded. Participants in each hospital were examined by the same trained doctors. Only first visit participants were included and divided into four groups according to their sampling regions (i.e., steel, coal, oil, and living). The study was performed in accordance with the Declaration of Helsinki and was approved by the Peking University Third Hospital Ethics Committee (Research Ethics Number M2019101). Informed consent was obtained from all participants.
Outdoor air pollutants and meteorology data
During the observation period, the daily mean temperature, relative humidity, and wind speed were provided by the meteorological administrations of Beijing, Hebei, Heilongjiang, Anhui, and Inner Mongolia. The concentrations of daily air quality index (AQI), PM2.5, PM10, O3, NO2, and SO2 were obtained from open-access government air-quality monitoring data. Since the air pollutants exposure are constant, the 24-h average concentrations of PM2.5, PM10, NO2, and SO2 as well as the 8-h maximum value of O3 were collected as daily exposures according to previous studies18,19,20,21. AQI is determined by monitoring the five major air pollutants, i.e., PM, O3, NO2, SO2 and carbon monoxide (CO), and the maximum value among each of the pollutants is assigned as the date- and location-specific AQI value20,21. As Gope et al.20 and Mirabelli et al.21 reports, we recorded 24-h average AQI as daily AQI. According to different AQI levels (level I: 0–50; level II: 51–100; level III: 101–150), participants were divided into three groups. The daily exposure is considered as short-term exposure. And we recorded the 1-month average AQI (AQI mean) before the first observation as long-term exposure.
Ocular surface health assessment
Symptoms were assessed using the ocular surface disease index (OSDI) questionnaire22. Lid margin morphology, MG morphology/function, tear meniscus height (TMH), Schirmer's test (ST), tear breakup time (TBUT), and CFS were examined in the individuals' right eyes, following previously reported methods23,24,25. Palpebral margin (hyperemia/telangiectasia, debris, edema/thickening, irregularity, and neovascularization) and MG (plugging, stenosis, expression secretion, and loss) were examined with the slit-lamp microscope. The MG loss was graded as follows26: 0 (no dropout), 1 (< 1/3 total area dropout), 2 (1/3–2/3 total area dropout), and 3 (> 2/3 total area dropout). The MG secretion was scored as follows27: 0 (clear meibum), 1 (cloudy meibum), 2 (granular meibum with debris), and 3 (inspissated meibum and toothpaste-like). MG expression was evaluated in five glands on the temporal, central, and nasal eyelid using the following standard: 0 (all glands expressible), 1 (three to four glands expressible), 2 (one to two glands expressible), and 3 (no glands expressible)17.
Tear film collection and cytokine measurement
Tear samples were collected from the right eyes of participants. Without any anesthetic, non-irritating tear collection was conducted using 5-μL capillary pipettes. A plastic head was used to squeeze tears into 0.2 mL Eppendorf tubes, and tears were immediately frozen at − 80 °C. The cytokine levels in the tear samples were measured by a flow cytometer (BD FACS Canto ll, Becton Dickinson, Franklin Lakes, NJ, USA) and a bead array system (BD Cytometric Bead Array system, Becton Dickinson) following the instructions of the manufacturer. At least 50 μL undiluted tear samples were analyzed for cytokines including interleukin 1 beta (IL-1β), interleukin 6 (IL-6), interleukin 8 (IL-8), interleukin 10 (IL-10), interleukin 17 (IL-17), tumor necrosis factor-alpha (TNF-α), interferon-gamma (IFN-γ), vascular endothelial growth factor (VEGF), and B-cell activating factor (BAFF).
Statistical analysis
Continuous variables were presented as the mean ± standard deviation (SD)/median (25% quantile, 75% quantile). Categorical variables were expressed as frequencies and percentages. Continuous variables were compared using analysis of variance or Kruskal–Wallis tests among four groups. Continuous data between two groups were compared using independent t tests or Mann–Whitney nonparametric U tests. Categorical variables were compared using the Chi-square test. Crosstab analyses were used to compare the status of the lid margin and MG morphology/function. Spearman correlation analysis was conducted to assess the associations between different atmospheric environments, various indices of ocular surface, and cytokine levels. Logistic regression analysis and multivariate linear regression analysis were used to evaluate the changes in ocular surface parameters and tear cytokines according to each air pollutant and meteorological variables. After variables collinearity checking and covariates (such as age and sex) being adjusted, regression models were developed. Since the MG function, conjunctivochalasis, OSDI, CFS and cytokine concentrations did not show a normal distribution, normality transition was performed before analysis. Statistical analysis was performed using SPSS version 23.0 (IBM Corp., Armonk, New York, USA). A P value < 0.05 was considered significant for all comparisons.
Results
Between February 2020 and February 2021, 864 individuals were included, including 198 patients from steel region, 78 from coal region, 276 from living region, and 312 from oil region. The demographic characteristics in the four regions are shown in Table 1. The gender (P = 0.067) and age (P = 0.743) among the four regions showed no significant difference.
Different regions presented varying air quality
The meteorological factors and ambient pollutants varied greatly and presented large disparity across the four regions, as shown in Table 1 and Fig. 1. The temperature (°C) in oil region was significantly lower than other regions (median temperature in oil: − 3, steel: 20, coal: 17, living: 21, P = 0.000), but the concentrations of O3 (μg/m3) and SO2 (μg/m3) were significantly higher than other regions (median O3 in oil: 66, steel: 38, coal: 17, living: 43.5; median SO2 in oil: 16, coal: 15, steel: 9, living: 9; all P = 0.000). Relative humidity (%), AQI, PM2.5 (μg/m3), and PM10 (μg/m3) in coal region were significantly higher than the other regions (median relative humidity: 81.5, AQI: 78, PM2.5: 107, PM10: 160, all P = 0.000). The wind speed (level) in steel and oil region was significantly higher than coal and living regions (median in steel: 3, oil: 3.5, coal: 1, living: 2, P = 0.000), but NO2 concentration (μg/m3) in steel region was significantly lower than the other regions (median in steel: 26, oil: 36, living: 36, coal: 28, P = 0.000).
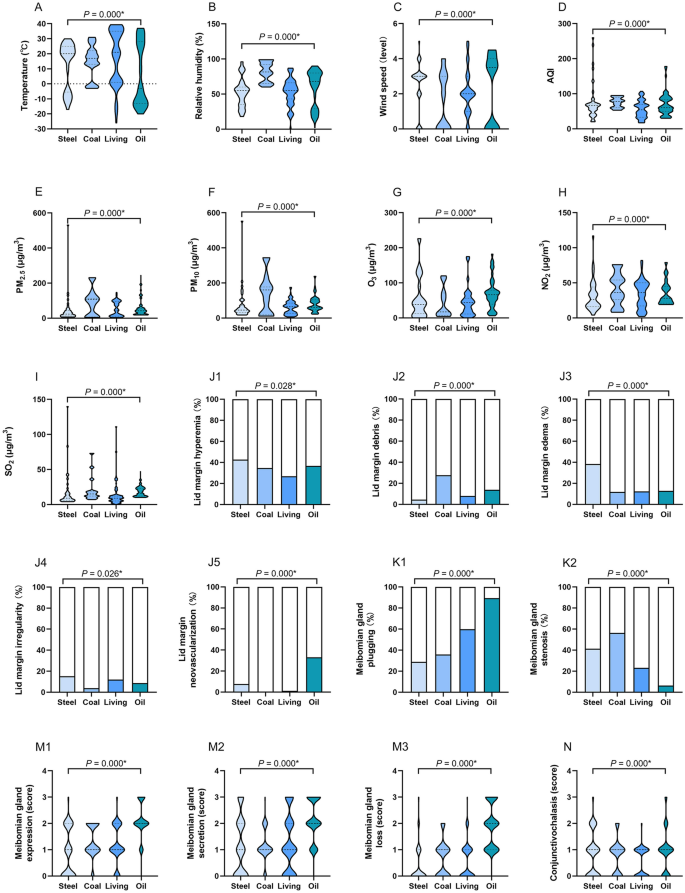
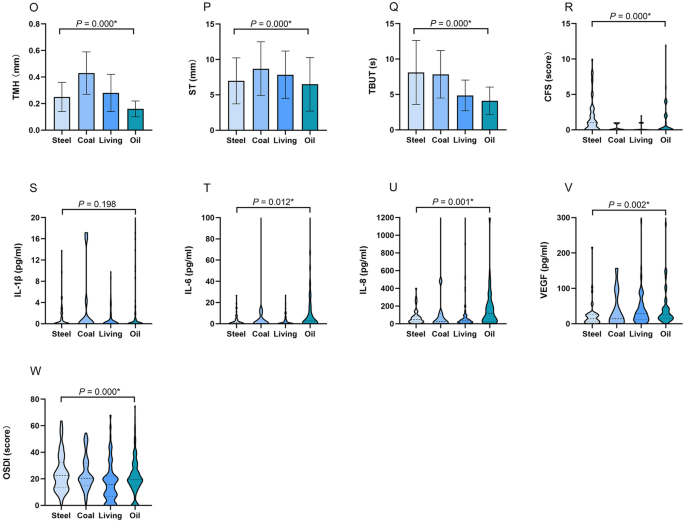
The differences of meteorological factors, ambient pollutants, ocular surface status and cytokine levels in four regions. In oil region, the temperature was significantly lower (A), the concentrations of O3 (G) and SO2 (I) were significantly higher than other regions. Relative humidity (B), AQI (D), PM2.5 (E), and PM10 (F) in coal region were significantly higher than other regions. The wind speed (C) in steel and oil region was significantly higher than coal and living regions. NO2 concentration (H) in steel region was significantly lower than other regions. Individuals in living region presented a low proportion of eyelid hyperemia (J1), coal and oil regions exhibited a high proportion of lid debris (J2). Participants in steel region had a relatively high proportion of lid edema (J3) and irregularity (J4). Individuals in oil and steel regions exhibited a high proportion of eyelid neovascularization (J5). Participants in oil region had a high incidence of MG plugging (K1), but few suffered from MG stenosis (K2). Individuals in oil region mainly exhibited higher levels of MG expression (M1), MG secretion (M2), MG loss (M3), and conjunctivochalasis (N) than other regions. Individuals in oil region demonstrated significantly lower TMH (O) and ST (P), shorter TBUT (Q), and higher CFS score (R), IL-6 (T), IL-8 (U), VEGF (V). Participants in living region reported less OSDI than other regions (W). The results were expressed as median with interquartile range in (A–I), (M,N) and (R–W); as percentage in (J–K); as mean ± standard deviation (SD) in (O–Q). AQI air quality index, PM particulate matter, O 3 ozone, NO 2 nitrogen dioxide, SO 2 sulfur dioxide, TMH tear meniscus height, ST Schirmer's I test, TBUT tear film break-up time, CFS corneal fluorescein staining, IL-1β interleukin 1 beta, IL-6 interleukin 6, IL-8 interleukin 8, VEGF vascular endothelial growth factor, OSDI ocular surface disease index, *P < 0.05.
Individuals in four regions presented different ocular surface status and cytokine levels
The ocular surface status and cytokine levels of individuals according to the four regions are shown in Table 1 and Fig. 1. More than half of the participants demonstrated a variety of lid margin abnormalities. Individuals in coal and oil regions exhibited a high proportion of lid margin debris (coal: 27.6%, oil: 13.8%, living: 8.0%, steel: 4.5%, P = 0.000). Individuals in oil and steel regions exhibited a high proportion of eyelid neovascularization (oil: 33.0%, steel: 7.6%, living: 1.1%, coal: 0%, P = 0.000). Participants in steel region had a relatively high proportion of lid edema/thickening (38.4%, P = 0.000) and irregularity (15.2%, P = 0.026). Individuals in living region presented a low proportion of eyelid hyperemia (living: 26.8%, coal: 34.6%, oil: 36.7%, steel: 42.7%, P = 0.028).
The abnormalities of MG morphology in the four regions demonstrated a difference, particularly in oil region. Compared with other regions, participants in oil region had a high incidence of MG plugging (oil: 89.4%, living: 59.8%, coal: 35.9%, steel: 28.8%, P = 0.000), but few suffered from MG stenosis (oil: 6.4%, living: 23.2%, steel: 41.4%, coal: 56.4%, P = 0.000). Regarding MG expression, MG secretion, MG loss, and conjunctivochalasis, individuals in oil region mainly exhibited higher levels than other regions (all P = 0.000).
Similar with lid margin and MG evaluation, individuals in oil region demonstrated significantly lower TMH (oil: 0.16 ± 0.06, steel: 0.25 ± 0.11, coal: 0.43 ± 0.16, living: 0.28 ± 0.14, P = 0.000) and ST (oil: 6.49 ± 3.79; steel: 6.98 ± 3.25, coal: 8.70 ± 3.81, living: 7.85 ± 3.35, P = 0.000), shorter TBUT (oil: 4.10 ± 1.95, steel: 8.12 ± 4.51, coal: 7.85 ± 3.36, living: 4.86 ± 2.17, P = 0.000), and higher CFS score (oil: 0 [0, 2], steel: 1 [0, 2.5], coal: 0 [0, 1], living: 0 [0, 0.25], P = 0.000). In terms of OSDI, participants in living region reported less discomfort than other regions (median in living: 15.91, steel: 22.55, coal: 20.45, oil: 19.32, P = 0.000).
Regarding cytokines to be tested, IL-10, IL-17, INF-γ, and TNF-α were not detected. Individuals in oil and coal regions exhibited the highest levels of IL-1β (oil: 0 [0, 1.35], coal: 0 [0, 3.26], steel: 0 [0, 1.25], living: 0 [0, 0], P = 0.198). Additionally, participants in oil region demonstrated the highest levels of IL-6, IL-8 and VEGF (median IL-6 in oil: 2.66, steel: 0.96, coal: 0.38, living: 0.56, P = 0.012; IL-8 in oil: 117.52, steel: 46.94, coal: 26.89, living: 33, P = 0.001; VEGF in oil: 25.09, steel: 14.02, coal: 14.02, living: 28.47, P = 0.002).
The diversity of ocular surface status in different regions was associated with air quality indices
The associations between air quality and MGD signs are shown in Table 2. In this logistic regression model, PM2.5, O3 and SO2 exposure showed an odd ratio (OR) 1.016 [95% confidence interval (CI) 1.004–1.038], OR 1.024 (95% CI 1.004–1.045), OR 1.037 (95% CI 1.015–1.059) for lid margin neovascularization, respectively (P = 0.043, P = 0.016, P = 0.001). PM10 exposure had an OR 1.017 (95% CI 1.002–1.031) for lid margin debris (P = 0.025). Temperature presented an OR 1.060 (95% CI 1.041–1.080) for lid margin hyperemia (P = 0.000), OR 1.037 (95% CI 1.007–1.068) for debris (P = 0.016), OR 1.040 (95% CI 1.009–1.073) for edema (P = 0.012), OR 0.934 (95% CI 0.904–0.965) for neovascularization (P = 0.000) and OR 0.965 (95% CI 0.948–0.982) for MG plugging (P = 0.000). Relative humidity had an OR 0.967 (95% CI 0.942–0.993) for lid margin irregularity (P = 0.012) and OR 0.977 (95% CI 0.963–0.992) for MG stenosis (P = 0.002). Age presented an OR 1.017 (95% CI 1.001–1.032) for lid margin hyperemia (P = 0.039), OR 1.097 (95% CI 1.051–1.146) for debris (P = 0.000), OR 1.107 (95% CI 1.045–1.171) for edema (P = 0.000), OR 1.094 (95% CI 1.044–1.147) for neovascularization (P = 0.000) and OR 1.016 (95% CI 1.003–1.029) for MG plugging (P = 0.015). No significant association of gender, wind speed, AQI and NO2 with MGD signs was observed.
The effects of air quality on ocular surface and tear cytokines are shown in Table 3. Multicollinearity between all air pollution variables was examined by ensuring that the variance inflation factors did not exceed 10. Significant associations were found between increased OSDI scores and higher PM2.5 [β = 0.146 (95% CI 0.094–0.198), P = 0.000, per 1 μg/m3 increase], higher PM10 [β = 0.118 (95% CI 0.080–0.156), P = 0.000, per 1 μg/m3 increase], higher O3 [β = 0.233 (95% CI 0.179–0.353), P = 0.020, per 1 μg/m3 increase], and higher SO2 [β = 0.137 (95% CI 0.093–0.210), P = 0.000, per 1 μg/m3 increase]. Significant associations were shown between decreased TMH and higher PM2.5 [β = − 0.074 (95% CI − 0.110 to − 0.012), P = 0.031] and higher O3 [β = − 0.075 (95% CI − 0.127 to − 0.010), P = 0.000]. Higher O3 exposure also associated with decreased ST [β = − 0.112 (95% CI − 0.145 to − 0.010), P = 0.043]. Decreased TBUT showed associations with higher PM2.5 [β = − 0.023 (95% CI − 0.032 to − 0.014), P = 0.000], higher O3 [β = − 0.024 (95% CI − 0.039 to − 0.010), P = 0.001] and higher SO2 [β = − 0.077 (95% CI − 0.092 to − 0.051), P = 0.000]. Significant associations were shown between increased CFS scores and higher PM2.5 [β = 0.021 (95% CI 0.013–0.028), P = 0.000], higher PM10 [β = 0.012 (95% CI 0.007–0.018), P = 0.000], higher O3 [β = 0.028 (95% CI 0.018–0.039), P = 0.001] and higher SO2 [β = 0.089 (95% CI 0.054–0.123), P = 0.000]. MG loss was associated with higher PM2.5 [β = 0.015 (95% CI 0.011–0.020), P = 0.000] and higher O3 [β = 0.015 (95% CI 0.010–0.020), P = 0.000]. However, no association was found between air pollutants and MG expression and secretion. PM was associated with tear cytokines, such as PM2.5 and IL-6 [β = 0.015 (95% CI 0.001–0.028), P = 0.035], IL-8 [β = 0.016 (95% CI 0.004–0.029), P = 0.013] and VEGF [β = 0.012 (95% CI 0.002–0.022), P = 0.044]; PM10 and IL-6 [β = 0.018 (95% CI 0.006–0.032), P = 0.006]. Higher O3 exposure was associated with increased IL-1β [β = 0.011 (95% CI 0.000–0.022), P = 0.045], IL-6 [β = 0.576 (95% CI 0.386–0.702), P = 0.007] and IL-8 [β = 0.479 (95% CI 0.369–0.890), P = 0.022], however, was not associated with VEGF concentrations. Increased VEGF was associated with higher NO2 [β = 0.012 (95% CI 0.003–0.022), P = 0.012] and higher SO2 [β = 0.021 (95% CI 0.001–0.047), P = 0.047]. Decreased temperature was associated with lower TBUT [β = 0.035 (95% CI 0.019–0.051), P = 0.001] and serious MG secretion [β = − 0.010 (95% CI − 0.016 to − 0.004), P = 0.000]. Because of collinearity, the AQI were not included in the analysis model.
The correlations between cytokine concentrations and ocular surface status during short-term exposure are shown in Table 4. Notably, the IL-6 concentration was moderately correlated with conjunctivochalasis (r = 0.312, P = 0.000), CFS (r = 0.307, P = 0.038), and TBUT (r = − 0.323, P = 0.000). The VEGF concentration was moderately correlated with CFS (r = 0.323, P = 0.028).
Air quality index monthly average was related to MGD
Participants were divided into three groups according to the average AQI 1 month before the study, resulting in 144 individuals in level I, 574 in level II, and 146 in level III. The 1-month average AQI (AQI mean) of levels I, II, and III were 38.95 ± 8.86, 77.34 ± 10.59, and 108.99 ± 8.76, respectively. The ocular surface status and cytokine levels of the three groups, and the correlations between the ocular surface and AQI mean during long-term exposure are shown in Table 5 and Fig. 2.
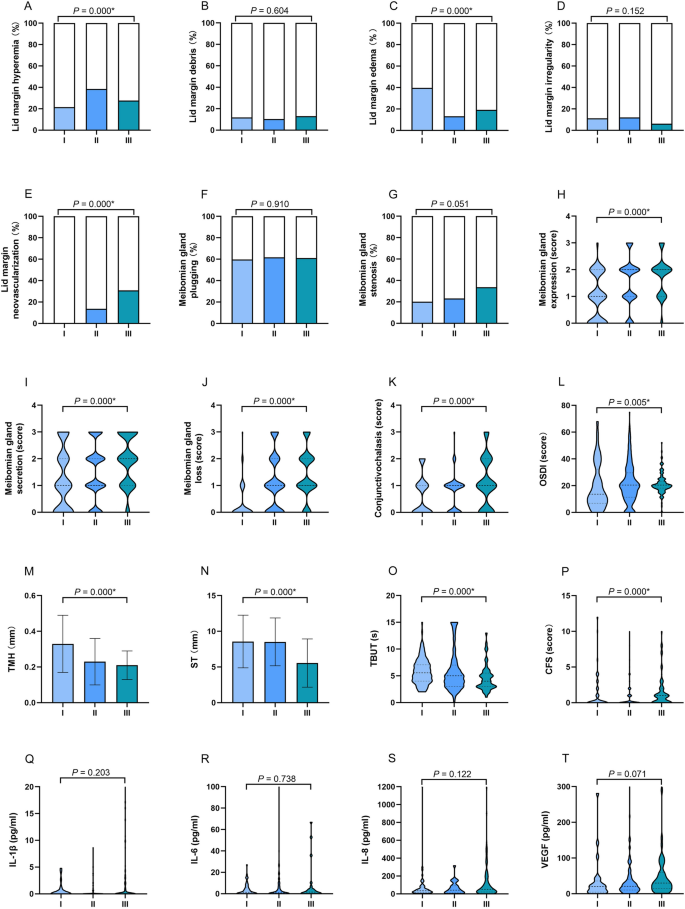
The ocular surface status and cytokine levels of the AQI level groups during long-term exposure. Individuals in Level II group exhibited a high proportion of lid hyperemia (A), Level I group exhibited a high proportion of eyelid edema (C), Level III group demonstrated a relatively high proportion of eyelid neovascularization (E), higher levels MG expression (H), MG secretion (I), MG loss (J), and conjunctivochalasis (K) than those in other groups. Individuals in Level III group had a significantly higher OSDI (L), lower TMH (M) and ST (N), shorter TBUT (O), and higher CFS score (P). The concentrations of IL-1β, IL-6, IL-8, and VEGF in three groups showed no difference. The results were expressed as percentage in A-G; as median with interquartile range in (H–L) and (O–T); as mean ± standard deviation (SD) in (M,N). AQI levels I: 0–50, II: 51–100, III: 101–150; OSDI ocular surface disease index, TMH tear meniscus height, ST Schirmer's I test, TBUT tear film break-up time, CFS corneal fluorescein staining, IL-1β interleukin 1 beta, IL-6 interleukin 6, IL-8 interleukin 8, VEGF vascular endothelial growth factor, *P < 0.05.
Individuals in Level II group exhibited a high proportion of lid hyperemia (II: 38.5%, III: 27.6%, I: 21.53%, P = 0.000). Individuals in Level I group exhibited a high proportion of eyelid edema (I: 39.6%, III: 19.2%, II: 13.1%, P = 0.000). Participants in Level III group demonstrated a relatively high proportion of eyelid neovascularization (III: 30.8%, II: 13.6%, I: 0%, P = 0.000). Regarding MG expression, MG secretion, MG loss, and conjunctivochalasis, individuals in level III group exhibited higher levels than those in other groups (all P = 0.000). Similar with eyelid and MG evaluation, individuals in Level III group had a significantly higher OSDI (median in III: 20.45, II:20.45, I: 13.62, P = 0.005), lower TMH (III: 0.21 ± 0.08, II: 0.23 ± 0.13, I: 0.33 ± 0.16, P = 0.000) and ST (III: 5.55 ± 3.38, II: 8.52 ± 3.35, I: 8.57 ± 3.69, P = 0.000), shorter TBUT (III: 4.65 ± 2.49, II: 5.88 ± 3.74, I: 5.84 ± 2.40, P = 0.000), and higher CFS score (median in III: 1, II: 0, I: 0, P = 0.000), Individuals in Level III group exhibited the highest concentrations of IL-1β, IL-6, IL-8, and VEGF. However, the difference was not significant.
Eyelid neovascularization (r = 0.402), MG expression (r = 0.377), MG secretion (r = 0.303), MG loss (r = 0.404), and TMH (r = − 0.345) were moderately correlated with AQI mean (P < 0.01).
Discussion
In this study, MGD signs (eyelid neovascularization, MG plugging, MG expression, MG secretion, and MG loss) in the participants from oil region were more frequent and severe compared with other regions. At the same time, individuals in oil region showed a significant reduction in the TMH, ST, and TBUT, and a significant increase in CFS, IL-1β, IL-6, IL-8, and VEGF concentration. In the long term, individuals exposed to higher AQI levels presented with MGD more commonly.
At present, many studies have reported that DED is related to air pollution28. As Mo et al. reported, PM2.5, PM10, SO2, NO2, and CO are strongly related with DED, while O3 was not18. However, Hwang et al. suggests that higher O3 and lower humidity were associated with DED in Korean, while PM10 was not29. NO2 and PM2.5 were also found to cause tear film stability and osmolality reduction30. Another study by Um et al. found that only SO2 was associated with DED in South Korea, while NO2, O3, CO, and PM10 were not31. Nevertheless, the impact of air pollutants on ocular surface remains controversial. In our study, lower temperature, higher O3, and higher SO2 levels in oil region are theorized to be contributing factors. Our result suggested that reduced temperatures might have favored the lower TBUT, more serious lid margin neovascularization, MG plugging and MG secretion. A possible reasonable explanation is that low temperatures may cool down the MG orifice surface, which results in the transient blockages of orifices, premature condensation of secretions and an increase in the viscosity after being discharged from MG orifices, leading to tear film instability and serious MGD signs32. However, the more serious lid margin hyperemia, debris and edema were seen in a relatively higher temperatures, suggested that elevated temperatures might favor the lid margin abnormality and inflammation. The results seemed to be a paradox. A human study was similar with our results that showing increased lipid layer thickness and TBUT but a high tear evaporation rate at a higher temperature32. Therefore, appropriate temperature is recommended for ocular surface health. O3 possesses the property of powerful oxidation and could affect the eye, skin, and respiratory system. Even at low concentrations, exposure to environmental O3 directly can be hazardous. A cross-sectional study from Korea suggested that higher O3 levels were strongly related to DED29. According to Lee et al., long-term exposure to a high concentration of O3 induces oxidative damage, enhances inflammatory cytokine levels in tears, and decreases tear production and conjunctival goblet cell density, known to be via the NF-κB pathway in vitro33,34. Additionally, in SOD1 (−/−) mice, increased periglandular inflammatory infiltrates, increased fibrosis, decreased glandular acinar density, and apoptosis were found in MGs35. Those previous researches in agreement with our results, the increased O3 concentration might contribute to the elevated cytokine levels (IL-1β, IL-6, IL-8), decreased ST, TMH and TBUT, higher OSDI and CFS scores, and serious MGD signs (MG loss and margin vascularization). Ozone is such a small molecule that it may approach the ocular surface and lacrimal glands, then induce inflammation and tear secretion abnormality. According to the studies of Wolkoff et al., aggressive aerosols and combustion products, such as SO2, could alter the structural composition of the outermost lipid layer of the precorneal tear film and cause eye burning, drying, and itching, resulting in DED in most cases15. The results of this study demonstrated SO2 concentrations were associated with more serious MGD (lid vascularization), lower TBUT, higher OSDI and CFS scores, and higher VEGF concentration, which is in accordance with the previously reported findings. Therefore, it is speculated that lower temperature induces temporary blockages of orifices, SO2 alters the lipid layer of the tear film, and excessive oxidative stress exacerbates inflammation and the chance of microbial infection.
In this study, individuals in oil regions presented more frequent and severe MGD, along with an increased level of cytokines, including IL-1β, IL-6, IL-8, and VEGF. Cytokine fluctuations were significant associated with air pollutants, such as PM, O3, and SO2 levels. The fluctuations in the cytokine concentrations showed a strong correlation with ocular surface changes, including conjunctivochalasis, TBUT and CFS, among others. These indicated that the expression of proinflammatory cytokines was sensitive to air pollutant changes, especially gases. Solomon et al. have detected an increase in the proinflammatory forms of IL-1 (IL-1α and mature IL-1β) in the tear fluid of patients with DED, suggesting that IL-1 may play a key role in the pathogenesis of keratoconjunctivitis sicca36. Barton et al. demonstrated an increased expression of IL-1, IL-6, and IL-8, and decreased epidermal growth factor levels in eyes with Sjögren's syndrome37. Lee et al. demonstrated that the expression of IL-6, IL-8, IL-17, and IFN-γ increased after exposure to O3 in cultured human conjunctival epithelial cells not pretreated with IL-1α34. Generally, IL-1β, IL-6, and IL-8 are considered to induce an oxidative stress response34. IL-1 and IL-6 jointly promote Th-17 cell differentiation, and IL-6 is also associated with the B-cell activation, proliferation, and differentiation34. The chronic inflammation is associated with high tear cytokines concentrations38,39,40, and both of them are important in the pathogenesis of MGD41. Therefore, the tear cytokines may play key roles in the pathogenesis of MGD exposed on air pollution.
According to the WHO, PM concentration in China have reached "bad" or even "very bad" levels, particularly in those industrial and densely populated areas. PM is identified as a crucial indicator of environment pollution and should draw public attention19,42. Previous studies demonstrated that high levels of PM2.5 and PM10 were associated with decreased TBUT and conjunctival goblet cells density and increased pro-inflammatory cytokines in mice30,43, which were in accordance with our results. There were some correlations between PM concentration fluctuations and MG morphology/function and tear quantity/stability. These results suggested that PM2.5 and PM10 were positively related to the severity of ocular surface pathologies and MGD, we suspect that the PM may first affect the MG, leading to abnormal morphology and secretory function, thus affecting the tear film stability and ocular surface, resulting in ocular surface discomfort. Fu et al. reported that PM2.5 has a time- and dose-dependent effect on cytotoxicity in cultured human corneal epithelial cells44. Thus, it may be difficult to detect ocular surface differences when the short-term changes in PM are not obvious. Yoon et al. have found that the effects of collected road dust on cellular responses are strongly dependent on their concentration and solubility45, indicating that atmospheric PM concentrations cannot be equal to PM concentrations in tears. Rather than PM concentrations, the components of PM are more important45,46. The specific compositions of PM, such as polyaromatic hydrocarbons, elemental/organic carbon, heavy metals, nitrate and sulfate47, change according to different zones and time. And the chemical characteristics of the compounds adsorbed to the particle surface will definite affect the PM toxic effects. In our study, the concentrations of PM2.5 and PM10 in the oil region were higher than those in the living and steel regions but lower than those in the coal region. However, the ocular surface presented more serious signs, and cytokine concentrations are higher than the three regions. We suspect that the air pollutants in the oil region are more soluble and complex, especially when affected by weather conditions, atmospheric chemistry, and complicated interactions with multiple air pollutants (such as O3 and SO2)47.
This study attempts to reflect the long-term pollution status by using the average preliminary AQI. Previous studies reported dose–response relationships in the constant concentration of air pollutants48,49. However, the exposure on the ocular surface is continuous, and we used the mean AQI in the present study inevitably. Symptoms and signs of MGD gradually increased with the 1-month average AQI, especially the lid margin neovascularization, MG expression, MG secretion, and MG loss. A certain transition period between the onset of DED and MGD signs is suspected, and long-term air quality likely plays an important role in the process. However, the AQI reflects the pollution levels of the most influential pollutants daily, which the 1-month AQI may not have captured.
The study had several limitations. First, the study's sample size was not large enough, making it difficult to stratify the differences in temperature and relative humidity for further analysis. Second, the study was a prospective cohort study, so the results did not definitively provide causal evidence between MGD and air pollutants. Third, the air pollutants exposure on the ocular surface is continuous. However, it is hard to monitor air quality personally and constantly in a large amount of people. We used the daily basis in the present study inevitably. There were differences between the indoor and outdoor activities of individual. However, our participants were asked to do 3–4 h outdoor activities to eliminate this difference. Despite some limitations, we believe this study is meaningful because it is a well-designed multicenter prospective study with organized statistical analysis. We investigate different air pollutants, ocular surface parameters and inflammation cytokines in different areas across China, and found it necessary to make a protocol to handle those air pollutants as much as possible to decrease their harmful effects.
In conclusion, individuals in the oil region presented more frequent and severe MGD, along with higher cytokine levels. MGD is likely in long-term exposure to relatively high AQI. The significant association between air pollution and ocular surface discomfort indicates the urgency to accelerate the environmental protection.
Data availability
The datasets used and/or analyzed during the current study are available from the corresponding author on reasonable request.
References
-
Sinharay, R. et al. Respiratory and cardiovascular responses to walking down a traffic-polluted road compared with walking in a traffic-free area in participants aged 60 years and older with chronic lung or heart disease and age-matched healthy controls: A randomised, crossover study. Lancet 391, 339–349. https://doi.org/10.1016/S0140-6736(17)32643-0 (2018).
-
Zhong, J. Y., Lee, Y. C., Hsieh, C. J., Tseng, C. C. & Yiin, L. M. Association between dry eye disease, air pollution and weather changes in Taiwan. Int. J. Environ. Res. Public Health 15, 2269. https://doi.org/10.3390/ijerph15102269 (2018).
-
Wang, X., Kindzierski, W. & Kaul, P. Air pollution and acute myocardial infarction hospital admission in Alberta, Canada: A three-step procedure case-crossover study. PLoS One 10, e0132769. https://doi.org/10.1371/journal.pone.0132769 (2015).
-
Su, C. et al. Short-term effects of fine particulate air pollution on cardiovascular hospital emergency room visits: A time-series study in Beijing, China. Int. Arch. Occup. Environ. Health 89, 641–657. https://doi.org/10.1007/s00420-015-1102-6 (2016).
-
Weichenthal, S., Lavigne, E., Evans, G., Pollitt, K. & Burnett, R. T. Ambient PM2.5 and risk of emergency room visits for myocardial infarction: Impact of regional PM2.5 oxidative potential: A case-crossover study. Environ. Health 15, 46. https://doi.org/10.1186/s12940-016-0129-9 (2016).
-
Mustafic, H. et al. Main air pollutants and myocardial infarction: A systematic review and meta-analysis. JAMA 307, 713–721. https://doi.org/10.1001/jama.2012.126 (2012).
-
Chan, S. H. et al. Long-term air pollution exposure and blood pressure in the sister study. Environ. Health Perspect. 123, 951–958. https://doi.org/10.1289/ehp.1408125 (2015).
-
Link, M. S. & Dockery, D. W. Air pollution and the triggering of cardiac arrhythmias. Curr. Opin. Cardiol. 25, 16–22. https://doi.org/10.1097/HCO.0b013e32833358cd (2010).
-
GBD. Global, regional, and national comparative risk assessment of 79 behavioural, environmental and occupational, and metabolic risks or clusters of risks, 1990–2015: A systematic analysis for the Global Burden of Disease Study 2015. Lancet 388, 1659–1724. https://doi.org/10.1016/S0140-6736(16)31679-8 (2015).
-
Meo, S. A. et al. Effect of environmental air pollution on type 2 diabetes mellitus. Eur. Rev. Med. Pharmacol. Sci. 19, 123–128 (2015).
-
Stafoggia, M. et al. Long-term exposure to ambient air pollution and incidence of cerebrovascular events: Results from 11 European cohorts within the ESCAPE project. Environ. Health Perspect. 122, 919–925. https://doi.org/10.1289/ehp.1307301 (2014).
-
Woodruff, T. J., Darrow, L. A. & Parker, J. D. Air pollution and postneonatal infant mortality in the United States, 1999–2002. Environ. Health Perspect. 116, 110–115. https://doi.org/10.1289/ehp.10370 (2008).
-
Jung, S. J., Mehta, J. S. & Tong, L. Effects of environment pollution on the ocular surface. Ocul. Surf. 16, 198–205. https://doi.org/10.1016/j.jtos.2018.03.001 (2018).
-
Pouyeh, B. et al. Impact of ocular surface symptoms on quality of life in a United States veterans affairs population. Am. J. Ophthalmol. 153, 1061–66.e3. https://doi.org/10.1016/j.ajo.2011.11.030 (2012).
-
Wolkoff, P. Ocular discomfort by environmental and personal risk factors altering the precorneal tear film. Toxicol. Lett. 199, 203–212. https://doi.org/10.1016/j.toxlet.2010.09.001 (2010).
-
Schaumberg, D. A. et al. The international workshop on meibomian gland dysfunction: Report of the subcommittee on the epidemiology of, and associated risk factors for, MGD. Investig. Ophthalmol. Vis. Sci. 52(4), 1994–2005. https://doi.org/10.1167/iovs.10-6997e (2011).
-
Tomlinson, A. et al. The international workshop on meibomian gland dysfunction: Report of the diagnosis subcommittee. Investig. Ophthalmol. Vis. Sci. 52, 2006–2049. https://doi.org/10.1167/iovs.10-6997f (2011).
-
Mo, Z. et al. Impacts of air pollution on dry eye disease among residents in Hangzhou, China: A case-crossover study. Environ. Pollut. 246, 183–189. https://doi.org/10.1016/j.envpol.2018.11.109 (2019).
-
Kim, Y., Choi, Y. H., Kim, M. K., Paik, H. J. & Kim, D. H. Different adverse effects of air pollutants on dry eye disease: Ozone, PM2.5, and PM10. Environ. Pollut. 265(Pt B), 115039. https://doi.org/10.1016/j.envpol.2020.115039 (2020).
-
Gope, S., Dawn, S. & Das, S. S. Effect of COVID-19 pandemic on air quality: A study based on Air Quality Index. Environ. Sci. Pollut. Res. Int. 28(27), 35564–35583. https://doi.org/10.1007/s11356-021-14462-9 (2021).
-
Mirabelli, M. C., Ebelt, S. & Damon, S. A. Air Quality Index and air quality awareness among adults in the United States. Environ. Res. 183, 109185. https://doi.org/10.1016/j.envres.2020.109185 (2020).
-
Michel, M., Sickenberger, W. & Pult, H. The effectiveness of questionnaires in the determination of contact lens induced dry eye. Ophthal. Physiol. Opt. 29, 479–486. https://doi.org/10.1111/j.1475-1313.2009.00658.x (2009).
-
Qiu, W. et al. Evaluation of the effects of conjunctivochalasis excision on tear stability and contrast sensitivity. Sci. Rep. 6, 37570. https://doi.org/10.1038/srep37570 (2016).
-
Pflugfelder, S. C. et al. Evaluation of subjective assessments and objective diagnostic tests for diagnosing tear-film disorders known to cause ocular irritation. Cornea 17, 38–56. https://doi.org/10.1097/00003226-199801000-00007 (1998).
-
Wolffsohn, J. S. et al. TFOS DEWS II diagnostic methodology report. Ocul. Surf. 15, 539–574. https://doi.org/10.1016/j.jtos.2017.05.001 (2017).
-
Arita, R., Itoh, K., Inoue, K. & Amano, S. Noncontact infrared meibography to document age-related changes of the meibomian glands in a normal population. Ophthalmology 115, 911–915. https://doi.org/10.1016/j.ophtha.2007.06.031 (2008).
-
Shimazaki, J., Goto, E., Ono, M., Shimmura, S. & Tsubota, K. Meibomian gland dysfunction in patients with Sjögren syndrome. Ophthalmology 105, 1485–1488. https://doi.org/10.1016/S0161-6420(98)98033-2 (1998).
-
Gupta, S. K. et al. A multicentric case–control study on the impact of air pollution on eyes in a metropolitan city of India. Indian J. Occup. Environ. Med. 11, 37–40. https://doi.org/10.4103/0019-5278.32463 (2007).
-
Hwang, S. H. et al. Potential importance of ozone in the association between outdoor air pollution and dry eye disease in South Korea. JAMA Ophthalmol. 134, 503–510. https://doi.org/10.1001/jamaophthalmol.2016.0139 (2016).
-
Torricelli, A. A. et al. Correlation between signs and symptoms of ocular surface dysfunction and tear osmolarity with ambient levels of air pollution in a large metropolitan area. Cornea 32, e11–e15. https://doi.org/10.1097/ICO.0b013e31825e845d (2013).
-
Um, S. B., Kim, N. H., Lee, H. K., Song, J. S. & Kim, H. C. Spatial epidemiology of dry eye disease: Findings from South Korea. Int. J. Health Geogr. 13, 31. https://doi.org/10.1186/1476-072X-13-31 (2014).
-
Abusharha, A. A., Pearce, E. I. & Fagehi, R. Effect of ambient temperature on the human tear film. Eye Contact Lens. 42(5), 308–312. https://doi.org/10.1097/ICL.0000000000000210 (2016).
-
Lee, H., Kim, E. K., Kim, H. Y. & Kim, T. I. Effects of exposure to ozone on the ocular surface in an experimental model of allergic conjunctivitis. PLoS One 12, e0169209. https://doi.org/10.1371/journal.pone.0169209 (2017).
-
Lee, H. et al. Effects of ozone exposure on the ocular surface. Free Radic. Biol. Med. 63, 78–89. https://doi.org/10.1016/j.freeradbiomed.2013.05.006 (2013).
-
Ibrahim, O. M. et al. Oxidative stress induced age dependent meibomian gland dysfunction in Cu, Zn-superoxide dismutase-1 (Sod1) knockout mice. PLoS One 9, e99328. https://doi.org/10.1371/journal.pone.0099328 (2014).
-
Solomon, A., Dursun, D., Liu, Z., Xie, Y. & Pflugfelder, S. C. Pro- and anti-inflammatory forms of interleukin-1 in the tear fluid and conjunctiva of patients with dry-eye disease. Investig. Ophthalmol. Vis. Sci. 42, 2283–2292 (2001).
-
Barton, K., Monroy, D. C., Nava, A. & Pflugfelder, S. C. Inflammatory cytokines in the tears of patients with ocular rosacea. Ophthalmology 104, 1868–1874. https://doi.org/10.1016/s0161-6420(97)30014-1 (1997).
-
Lam, H. et al. Tear cytokine profiles in dysfunctional tear syndrome. Am. J. Ophthalmol. 147(2), 198–205. https://doi.org/10.1016/j.ajo.2008.08.032 (2009).
-
Acera, A., Rocha, G., Vecino, E., Lema, I. & Durán, J. A. Inflammatory markers in the tears of patients with ocular surface disease. Ophthal. Res. 40(6), 315–321. https://doi.org/10.1159/000150445 (2008).
-
Kang, M. H. et al. Interleukin-17 in various ocular surface inflammatory diseases. J. Korean Med. Sci. 26(7), 938–944. https://doi.org/10.3346/jkms.2011.26.7.938 (2011).
-
Baudouin, C. et al. Revisiting the vicious circle of dry eye disease: A focus on the pathophysiology of meibomian gland dysfunction. Br. J. Ophthalmol. 100(3), 300–306. https://doi.org/10.1136/bjophthalmol-2015-307415 (2016).
-
Kim, K. H., Kabir, E. & Kabir, S. A review on the human health impact of airborne particulate matter. Environ. Int. 74, 136–143. https://doi.org/10.1016/j.envint.2014.10.005.40 (2015).
-
Torricelli, A. A. et al. Effects of ambient levels of traffic-derived air pollution on the ocular surface: Analysis of symptoms, conjunctival goblet cell count and mucin 5AC gene expression. Environ. Res. 131, 59–63. https://doi.org/10.1016/j.envres.2014.02.014 (2014).
-
Rückerl, R. et al. Air pollution and markers of inflammation and coagulation in patients with coronary heart disease. Am. J. Respir. Crit. Care Med. 173, 432–441. https://doi.org/10.1164/rccm.200507-1123OC (2006).
-
Yoon, S., Han, S., Jeon, K. J. & Kwon, S. Effects of collected road dusts on cell viability, inflammatory response, and oxidative stress in cultured human corneal epithelial cells. Toxicol. Lett. 284, 152–160. https://doi.org/10.1016/j.toxlet.2017.12.012 (2018).
-
Jia, Y. Y., Wang, Q. & Liu, T. Toxicity research of PM2.5 compositions in vitro. Int. J. Environ. Res. Public Health 14, 232. https://doi.org/10.3390/ijerph14030232 (2017).
-
Kelly, F. J. & Fussell, J. C. Size, source and chemical composition as determinants of toxicity attributable to ambient particulate matter. Atmos. Environ. 60, 504–526 (2012).
-
Li, J. et al. A mouse dry eye model induced by topical administration of the air pollutant particulate matter 10. Biomed. Pharmacother. 96, 524–534. https://doi.org/10.1016/j.biopha.2017.10.032 (2017).
-
Tan, G. et al. Air pollutant particulate matter 2.5 induces dry eye syndrome in mice. Sci. Rep. 8(1), 17828. https://doi.org/10.1038/s41598-018-36181-x (2018).
Funding
This work was supported by "National natural science foundation of Beijing" (No. 7202229). The funding organization had no role in the design or conduct of this research.
Author information
Authors and Affiliations
Contributions
R.H. and Y.W. setup the protocol and recruited the participants. R.H. collected and analyzed the data, created the figures, and contributed to the writing. Y.W. and L.Z. discussed the data and participated in writing manuscript. Y.L., M.S., J.D., Y.X., F.W., J.W. X.X., Z.L. and S.L. recruited the participants. X.L. setup the protocol and oversaw the final manuscript.
Corresponding author
Ethics declarations
Competing interests
The authors declare no competing interests.
Additional information
Publisher's note
Springer Nature remains neutral with regard to jurisdictional claims in published maps and institutional affiliations.
Rights and permissions
Open Access This article is licensed under a Creative Commons Attribution 4.0 International License, which permits use, sharing, adaptation, distribution and reproduction in any medium or format, as long as you give appropriate credit to the original author(s) and the source, provide a link to the Creative Commons licence, and indicate if changes were made. The images or other third party material in this article are included in the article's Creative Commons licence, unless indicated otherwise in a credit line to the material. If material is not included in the article's Creative Commons licence and your intended use is not permitted by statutory regulation or exceeds the permitted use, you will need to obtain permission directly from the copyright holder. To view a copy of this licence, visit http://creativecommons.org/licenses/by/4.0/.
Reprints and Permissions
About this article
Cite this article
Hao, R., Wan, Y., Zhao, L. et al. The effects of short-term and long-term air pollution exposure on meibomian gland dysfunction. Sci Rep 12, 6710 (2022). https://doi.org/10.1038/s41598-022-10527-y
-
Received:
-
Accepted:
-
Published:
-
DOI : https://doi.org/10.1038/s41598-022-10527-y
Source: https://www.nature.com/articles/s41598-022-10527-y
0 Response to "Hat is a Possible Longterm Effect of Continued Exposure to Air Pollution"
Post a Comment